
The Research Projects
TAVI-related Complications and Treatments
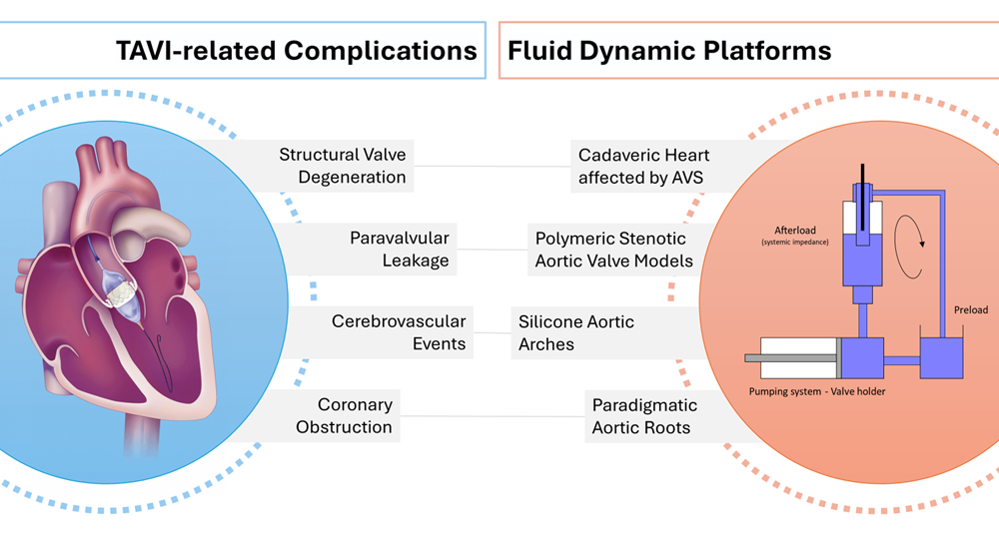
Transcatheter Aortic Valve Implantation (TAVI) represents a revolution in the treatment of aortic stenosis. By allowing the replacement of the degenerated native valve with a percutaneous valve, thereby avoiding the need for open-heart surgery, TAVI offers a valid alternative for high-risk patients for whom traditional surgery poses significant risks.
Despite the less invasive approach, TAVI is not without complications. Common issues include vascular injuries, stroke, paravalvular regurgitation, coronary occlusion – especially following Valve-in-Valve procedures – and structural valve degeneration, raising concerns about the long-term durability of the prosthesis. Managing these risks is crucial for the widespread adoption of TAVI.
In recent years, several new devices and techniques have been developed to address these complications. Fluid dynamic platforms have proven to provide a safe, controlled, and realistic environment to assess the hemodynamic performance of this new devices, allowing for repeatable and specific studies.
In this context, this research focuses on the design and development of fluid dynamic platforms tailored for transcatheter aortic procedures, aiming to investigate TAVI-related complications and facilitate the advancement of new strategies.
Soft Robotic Cardiac Simulator
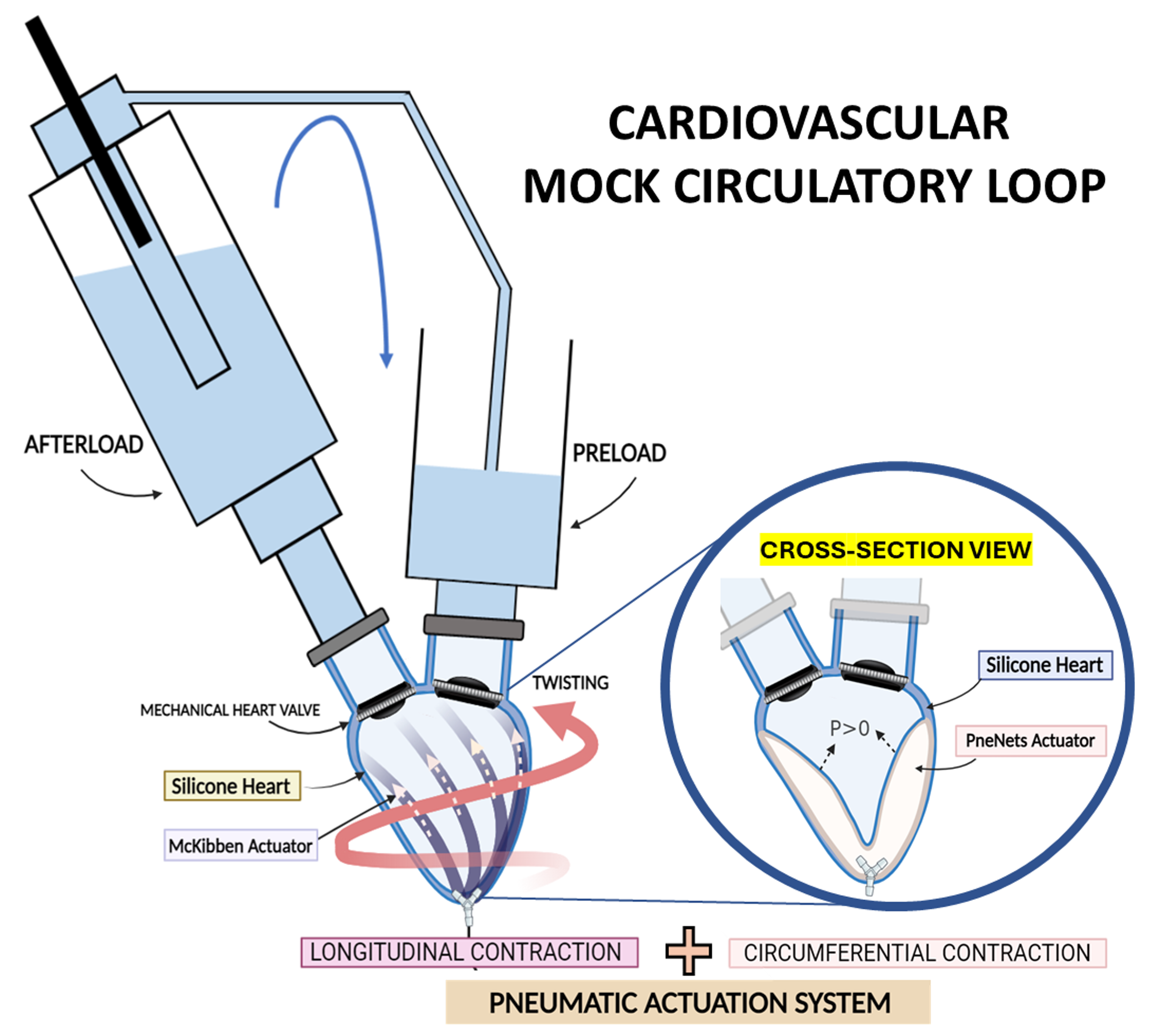
The aging global population and rising incidence of cardiovascular diseases have led to the spread of new transcatheter and minimally invasive cardiac procedures, offering a viable option for high-risk surgical patients. However, these procedures present significant challenges for clinicians, as devices interact with complex anatomies under dynamic heart conditions, relying primarily on X-ray fluoroscopy and echocardiography for guidance.
Consequently, there is a need to develop cardiac simulators not only to train clinicians in a safe, realistic, and controlled environment but also to provide valuable tools for testing medical devices and advancing research in cardiac interventions. This project will explore an innovative approach to designing and developing a patient-specific beating heart simulator integrated into a simulated circulatory system, utilizing soft robotic technology. The process will involve developing a patient-specific ventricle model, with active contraction achieved using soft pneumatic artificial muscles (PAMs) activated by air pressure. A detailed design study will integrate these PAMs to enable both circumferential and longitudinal contractions characteristic of natural heart muscles.
Furthermore, the cardiac model will undergo rigorous testing and integration into a dedicated cardiovascular mock-loop featuring polymeric valves. This setup will replicate cardiac phases, allowing for the evaluation of fluid dynamic parameters and ensuring a highly realistic simulation environment. Comprehensive testing protocols will be established to validate the integrated system’s performance and reliability across various operational scenarios.
Hybrid operating room mock loops
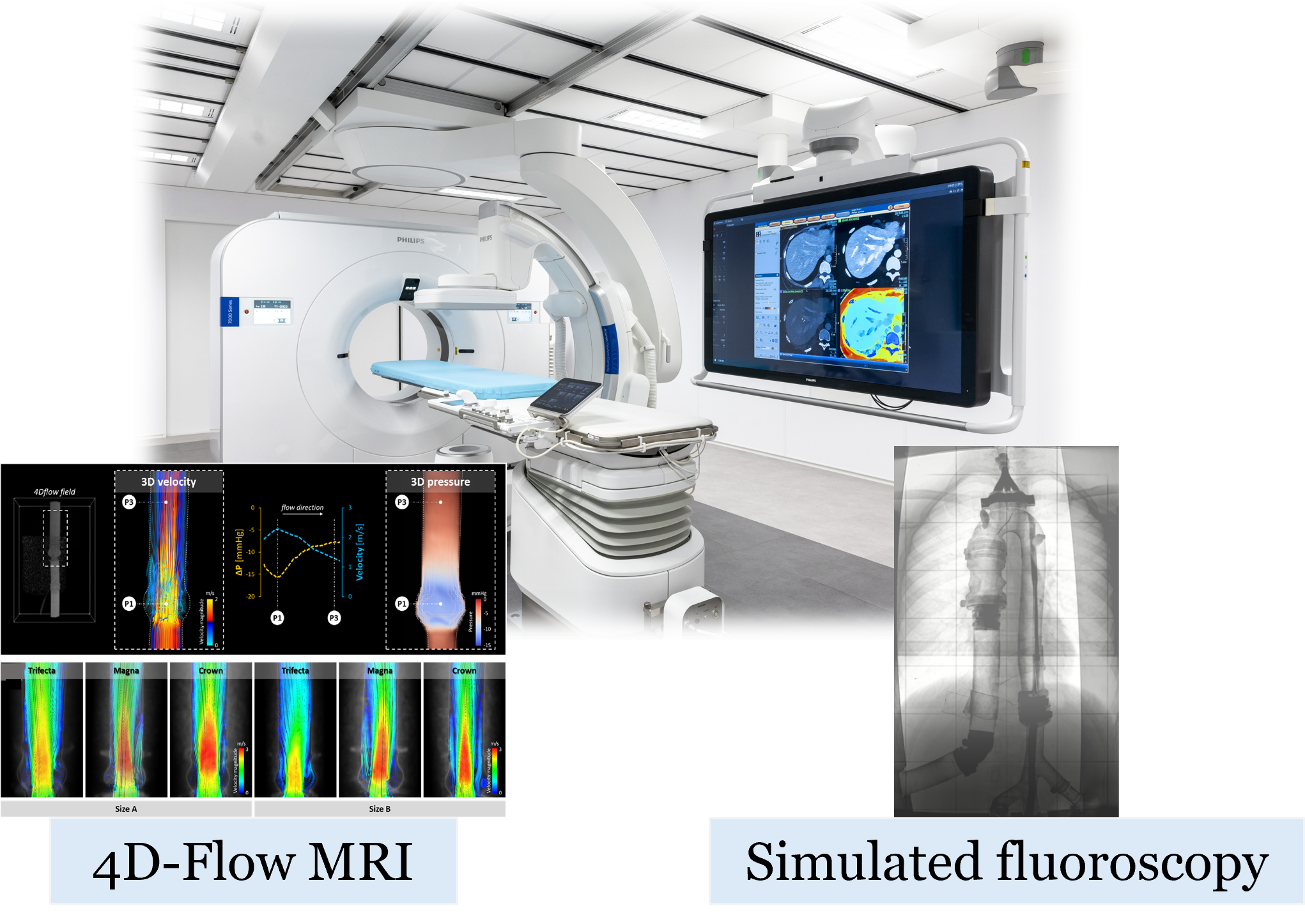
With the recent establishment of minimally invasive transcatheter procedures as gold standard to treat different cardiovascular diseases, imaging techniques, including X-ray fluoroscopy and magnetic resonance imaging (MRI), have assumed a crucial role for the diagnosis, evaluation, and intraprocedural monitoring during catheterization. Due to the lack of a clear and comprehensive view of the tissues of interest, the catheter, and the implantable device, clinicians and researchers have had to acquire new skills to integrate imaging in the daily clinical practice. In this context, the research activity aims to reproduce the hybrid operating room environment by developing experimental mock loops, capable of simulating in vivo physiological and pathological conditions, that are compatible with main imaging techniques. In particular, an MRI-compatible pulsatile mock loop for 4D flow MRI acquisitions and a mock loop for simulated X-rays fluoroscopy acquisitions will be developed.
Such platforms would provide clinicians with a primary tool for training, as they would allow not only to replicate the transcatheter procedure on a test bench and assess the procedure’s effectiveness from a hemodynamic perspective (through 4D-flow MRI acquisitions), but also to learn the correct setting of X-ray fluoroscopy and the interpretation of the associated images.
To this aim, the project will integrate four technologies: i) artificial intelligence for the automatic segmentation of pre-operative medical imaging; ii) mixed reality to intuitively navigate the image-based 3D anatomical model, to plan the procedure, and to visualize the planned scenario in the OR; iii) cloud computing to allow for fast reconstruction of the anatomical models and deplyment of the mixed reality app from GDPR-compliant platforms; iv) markerless hologram-to-patient registration algorithms allowing for precision without the need for bulky equipment or for cumbersome registration procedures. Our solution will be developed and tested in vitro on physical phantoms, and its usability will be tested in the OR.
Novel strategies for Tricuspid Valve treatment
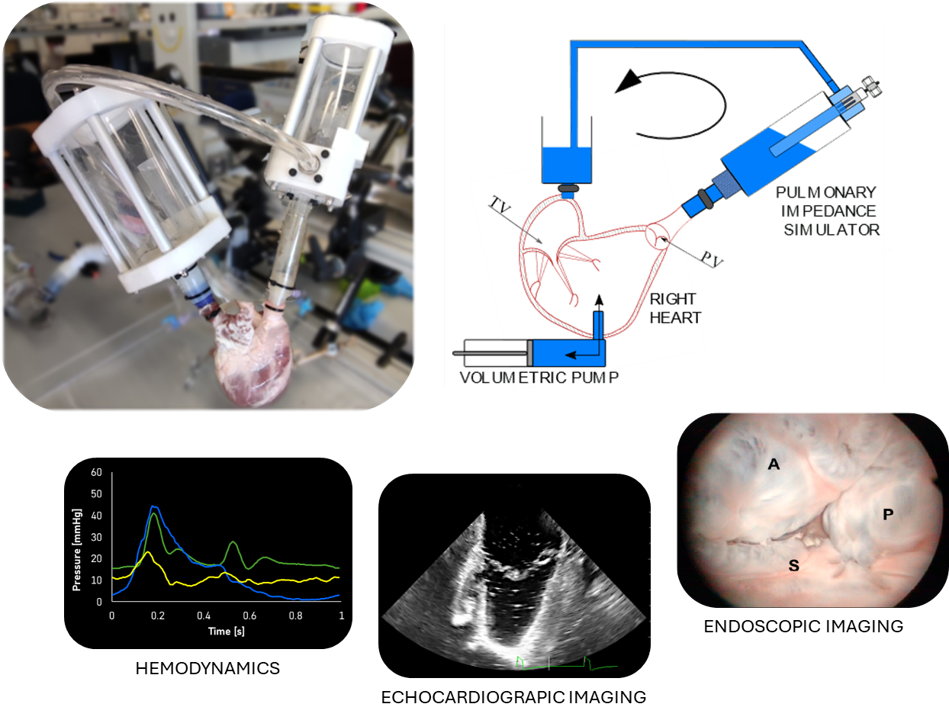
Tricuspid regurgitation (TR) is a pathology affecting 1.6 million patients in the US. It is characterized by blood leakage across the valve due to valve leaflet malcoaptation. Surgical open-heart TV repair is often avoided for patients in elderly ages or with comorbidities, so most of the patient is left untreated. Transcatheter devices represent a solution for vulnerable patient lowering operatory risks by reducing the invasiveness of the procedure. However, the novelty of the procedures demands new skills as from the clinicians as from the medical innovators during device development phases.
Reliable experimental platforms can be of help in accelerating the development of new therapies, novel devices and in defining new directions for heart valve treatments. At the same time, these platforms could provide hand-on high-fidelity training helping to reduce the occurrence of procedural errors and improve clinicians’ expertise.
In our laboratory, an ex vivo model of FTR is simulated through a pulsatile-flow mock loop of the pulmonary circulation using defrosted porcine right hearts. This model is able to reproduce the pathology from the hemodynamic and morphometric point of view and represents a well controllable environment for device assessment. The platform is compatible with echocardiographic and endoscopic imaging and can be equipped with force transducer to perform biomechanical evaluation of device interaction with the cardiac structures. During these years the platform was exploited for the preclinical validation of implantable devices helping innovators of medical industry with design feedback and with device performance evaluation.